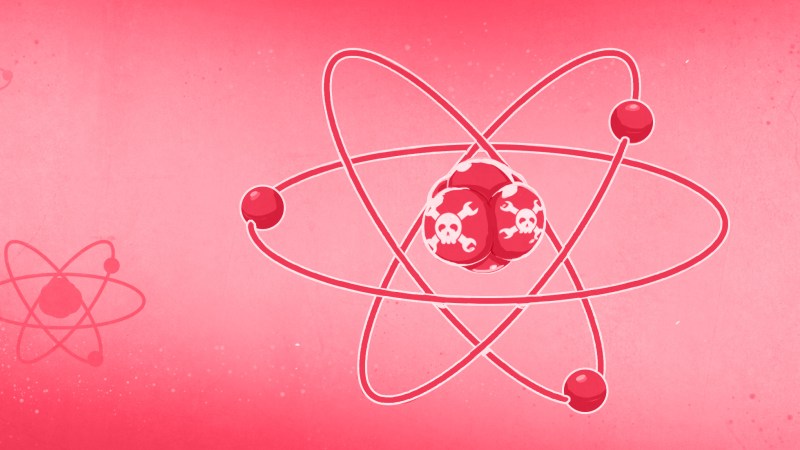
Particle physics is a field of extremes. Scales always have 10really big number associated. Some results from the Large Hadron Collider Beauty (LHCb) experiment have recently been reported that are statistically significant, and they may have profound implications for the Standard Model, but it might also just be a numbers anomaly, and we won’t get to find out for a while. Let’s dive into the basics of quantum particles, in case your elementary school education is a little rusty.
It all starts when one particle loves another particle very much and they are attracted to each other, but then things move too fast, and all of a sudden they’re going in circles in opposite directions, and then they break up catastrophically…
The Standard Model
In the 1970s physicists started coalescing around a thing called the Standard Model, which is similar to the Periodic Table of Elements, but at a much smaller scale. It describes the particles that make up the protons, neutrons, and electrons (which in turn make up atoms), and the forces that act on them. The Standard Model has held up to most experiments so far, but the one earlier this year may throw a small wrench in that.
The Standard Model reuses a lot of pre-existing words in confusing ways, so let’s break it down a little bit from (relatively) large to small.
- A molecule is made of atoms.
- An atom is made of protons and neutrons surrounded by electrons.
- Protons and neutrons are called composite particles because they’re made of smaller elementary particles.
- Protons and neutrons are made of combinations of quarks. There are other composite particles, and in general these particles made up of combinations of quarks are called hadrons.
- There are 6 different types of quarks, named: up/down, charm/strange, top/bottom. Combinations of up and down quarks make up protons and neutrons.
- In addition to quarks, there’s another class of particle called the lepton. Leptons can have a charge, like an electron, or they can not have a charge, like a neutrino.
Besides the classification of these tiny particles, the Standard Model also describes how the fundamental forces interact. There are 4; electromagnetism, strong nuclear, and weak nuclear. Gravity is the fourth, but the Standard Model doesn’t like to talk about this black sheep of a force, and intentionally leaves it as a blank space, an exercise for the reader (or some janitor to Good Will Hunting it). Some day either the force of gravity will be incorporated into the Standard Model, or a new model will emerge that explains the universe better than the Standard Model AND incorporates gravity, but until that happens, some hand-waving will occur. Besides, gravity works on such a larger scale than the other three, that its effect over the scale of subatomic particles is considered negligible. *shrug* I’m just repeating what the physicists are saying.
Keep Up with Bosons and Mesons
The way forces work is with force-carrying particles, a category called bosons. There are a few sub-categories here, because different bosons are responsible for the different forces. Photons and gluons are among them, and the recently discovered Higgs boson, which was theorized a long time ago as being a requirement for the Standard Model to work, so the physicists breathed a collective sigh of relief when it was finally found. There’s also a theorized graviton that would be the force-carrier for gravity, but it hasn’t been discovered… yet (looking at you, Matt Damon).
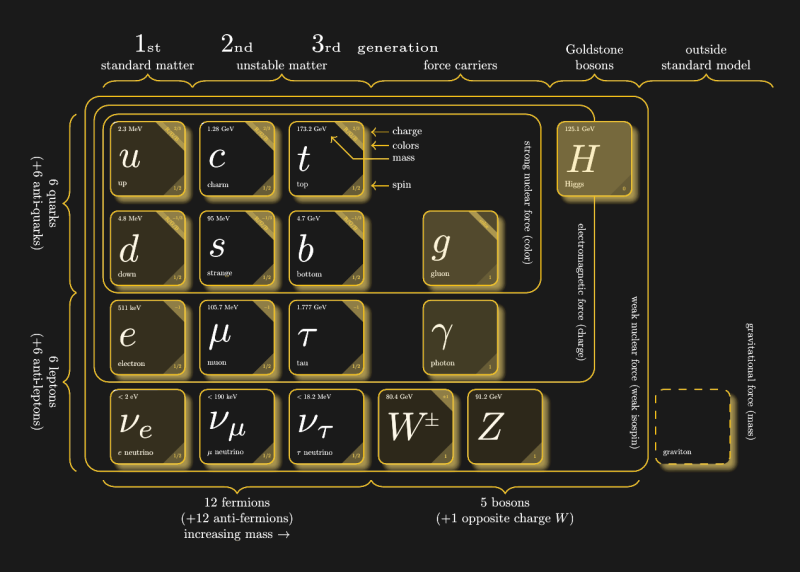
[CC-BY Carsten Burgard]
Clear?
The Experiment
Particles this small are impossible to measure directly, which is why we have the Large Hadron Collider. If one were to try to reverse engineer a cake, one could take pictures of it, do spectral analysis, maybe even taste it. These aren’t options at the subatomic level. Instead, the LHC makes particles move very fast, then bashes them against each other. The bits that result from these collisions have a lot of energy to dissipate, which results in them interacting with electromagnetic fields in tiny but measurable ways. From these collisions, we can work backwards to figure out the secrets of the universe, in much the same way that throwing a grenade at a cake and analyzing the spray pattern might allow you to determine whether the frosting was buttercream or fondant.
In the experiment announced recently (pdf of paper), there was a discrepancy in how a particular variant, the B meson (which isn’t a single variant but a whole class of variants), decayed. The Standard Model says that leptons all behave the same and are identical in every way except for their mass. So when the LHC bashed a whole bunch of particles together (specifically protons vs. protons) and measured the B meson decays, they theorized the subatomic soup that resulted would contain equal parts of electrons and muons. Instead they found 15% fewer muons than they expected.
They couldn’t exactly look at the nutrition label, so they’re left wondering if what they found was a fluke, or if the recipe was wrong. If it’s the former, then it will have beaten the odds by 3 sigma (which corresponds to a 1 in 740 chance of a fluke). In particle physics, this is merely eyebrow-raisingly interesting, as it’s not a large enough exponent to satisfy them. If it’s the latter, it means big things for the Standard Model. That could mean either revisions to the Standard Model, or possibly understanding if there’s a difference between the leptons (other than their mass alone), ending what was formerly called lepton universality.
Unfortunately the testing machine, much like a McDonald’s ice cream machine, is down for servicing, so we’ll have to wait until 2022 before the upgraded LHC can deliver some frosty mesons and give us an answer about the Standard Model.
Fool Me Once, Well, Keep Fooling Me
Calling this eyebrow-raisingly interesting as opposed to earth-shatteringly amazing is an appropriate response, as we’re no strangers to the teasings of the physicists. There have been a number of anomalies over the last decade in particle physics that have all been overturned eventually as more data came in. There’s a vast difference between a 3 sigma anomaly and a 5 sigma (1:3.5 million) discovery, and we’ve been seduced by this before. Maybe you remember in 2011 the superluminal neutrinos that turned out to be an improperly attached fiber optic cable. In 2015 there was the 750 GeV bump with a significance of 3.9 that ended up being a statistical fluctuation the next year when data was collected again. In 2016 there was an anomaly with the B meson that seems to have faded, too.
Each of these anomalies leads to hundreds of papers and theorizations and new types of physics until the next set of data sends them into the shredders to make pulp for the next round of papers, and the machine grinds on, with journalists siphoning headlines from these papers and drawing wild conclusions about warp speed and time travel and new particles like the leadingmeon.
And yet, forward progress keeps happening, slowly and scientific methodically, as we move towards understanding the workings of the universe. Maybe in the decades to come they’ll laugh at our quaint Standard Model like we look at the four elements of Earth, Wind, Air, and Fire, but we will have gotten to that point by moving through where we are today, so I’ll continue to read about it and nod like I pretend to understand what they’re talking about, and respect the complexities of the process of measuring things so small. After all, my day job as an electrical engineer relies on making electrons move in ways that were inconceivable a century ago; maybe the discovery of lepton non-universality will eventually lead to the downfall of Amazon and Uber.
0 Commentaires