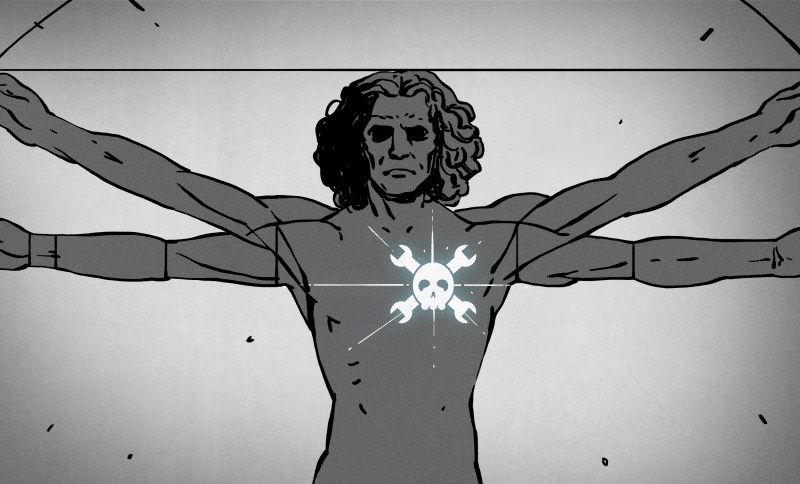
The number of artificial prosthetic replacement parts available for the human body is really quite impressive. From prosthetic eyes to artificial hips and knees, there are very few parts of the human body that can’t be swapped out with something that works at least as well as the original, especially given that the OEM part was probably in pretty tough shape in the first place.
But the heart has always been a weak spot in humans, in part because of the fact that it never gets to rest, and in part because all things considered, we modern humans don’t take really good care of it. And when the heart breaks down past the point where medicine or surgery can help, we’re left with far fewer alternatives than someone with a bum knee would face. The fact is that the best we can currently hope for is a mechanical heart that lets a patient live long enough to find a donor heart. But even then, tragedy must necessarily attend, and someone young and healthy must die so that someone else may live.
A permanent implantable artificial heart has long been a goal of medicine, and if recent developments in materials science and electrical engineering have anything to say about it, such a device may soon become a reality. Heart replacements may someday be as simple as hip replacements, but getting to that point requires understanding the history of mechanical hearts, and why it’s not just as simple as building a pump.
The Heartbeat of America
While the understanding of the heart as a pump stretches all the way back to the 3rd century BCE, it took nearly 1,000 more years for medical science to advance much; the early view of the Greek physician Galen that the heart provided heat for the body and blood moved from the venous to arterial systems via pores in the septum of the heart was not to be questioned, at least in Western medical traditions.
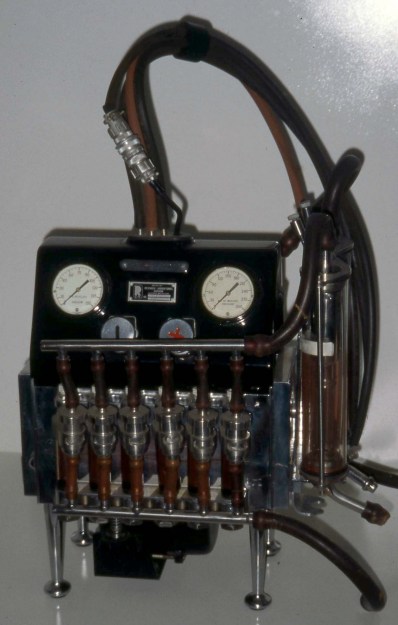
Once doctors were free to explore the human heart, its structure and function became clear. The heart is a four-chambered pump made from specialized muscle tissue. The upper chambers are referred to as the atria, feeding into larger ventricles below via one-way valves to prevent backflow. The right atrium receives deoxygenated blood from the body through a large vessel called the vena cava; it moves to the right ventricles and on toward the lungs, where through a complex process of gas exchange, it loses CO2 and gains oxygen. The oxygenated blood returns to the heart’s left atrium, and then into the powerful left ventricle, which supplies the entire body with oxygenated blood via the aorta.
Looking at the heart mechanistically, it’s easy to see why attempts at building a mechanical substitute for it extend all the way back to the 1930s. Soviet scientist Vladimir Demikhov was the first to try a mechanical heart, a device of his own design which he put into a dog. The animal lived for two hours after surgery.
Other surgeons used Demikhov’s dog experiments as the basis for human artificial hearts, first using extracorporeal devices like the Dodrill-GMR, a device built by car manufacturer General Motors Research. The Dodrill-GMR was used in 1952 to bypass the left ventricle of a 41-year-old patient during an operation to repair his mitral valve; he survived 50 minutes on the machine and ended up living for 30 more years.
Early experience with the Dodrill-GMR and follow-on heart-lung machines gave doctors valuable feedback on what works and what doesn’t work when it comes to pumping blood. After all, blood is not a simple fluid; it’s a complicated liquid tissue that is composed of blood cells (erythrocytes and leukocytes) as well as plasma. The heart is optimized to pump this fluid, introducing as little turbulence and shear forces as possible to keep the cells intact. Any mechanical substitute for the heart must not introduce such disruptive forces, which are liable to result in injury to the patient.
A Bridge Too Far
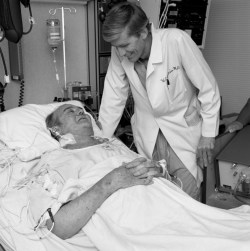
Early efforts at mechanical circulation focused on completely extracorporeal devices, acknowledging the fact that the engineering of the day was nowhere near ready to provide a totally implantable artificial heart. But the dream of a mechanical replacement heart lived on, mainly because the demand for healthy human hearts for transplant continued to outstrip supply. This became more and more the case as engineering for motor vehicles improved through the decades; the sad fact is that auto accidents provide the most reliable source of viable donor hearts, and as cars got safer, the donor pool got smaller.
The first so-called total artificial heart (TAH) to capture widespread attention was the Jarvik-7 heart, which was used for the first time clinically on Barney Clark, a retired dentist with severe congestive heart failure. Despite the fact that Clark had to be tethered to a large air compressor for the 112 days that he lived after surgery, the Jarvik-7 was the first artificial heart where the pumping mechanism was completely inside the chest cavity. It set the standard for what counts as a total artificial heart even today — the pumping mechanism is implantable, but due to engineering limitations, they have to be powered from outside the body.
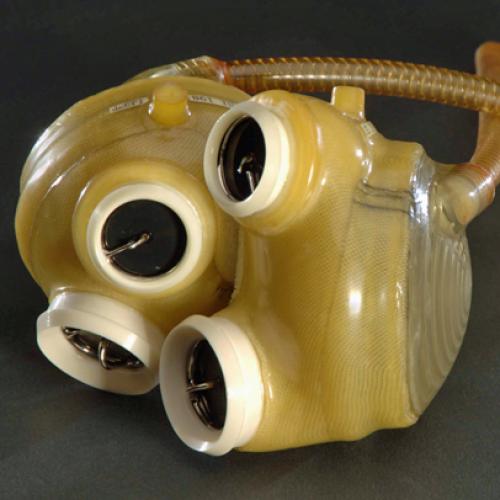
Both of the total artificial hearts currently approved for use in patients today rely on external power. The SynCardia TAH, a direct descendant of the Jarvik-7 heart made with better, more durable materials and equipped with valves that are less likely to damage blood cells, is still powered by compressed air, although the drive unit has been miniaturized enough that patients can go home with one. The other TAH, made by French company Carmat, uses a hydraulically driven flexible diaphragm to pump blood. The hydraulic power pack is small enough to be carried around, and is powered by Lithium-ion batteries.
Despite their mechanical differences, both these devices have something in common: they are not intended to be used as permanent replacement hearts, but rather as a bridge to buy some time for a donor heart to become available. The main reason for this is simple material science: it’s very hard to find materials that can flex continuously 70 to 80 times a minute for years on end without tearing. The best Carmat has managed to do is about 1,400 days, and while that’s more than ten times better than Barney Clark’s experience, it’s nowhere near enough. The other problem is that routing pneumatic or hydraulic lines into the thoracic cavity is clinically problematic, primarily through the risk of infection.
No Pulse, No Problem
While it might sound like a permanent TAH is still a long way off, there’s a lot going on in this space that might shorten the journey. A startup called BiVACOR is working on a design that’s vastly different than the SynCardia or Carmat hearts, and it contains components that will look very familiar to any hardware hacker. Rather than rely on diaphragms to move blood, the BiVACOR TAH used a centrifugal pump, whose impeller blades are directly in contact with the blood and provide a continuous flow. Normally, this would be an unacceptable source of shearing forces that would tear blood cells apart, not to mention the need for bearings which would eventually wear out. The BiVACOR TAH avoids these issues by magnetically levitating the impeller, leaving a wide gap between it and the pump housing. The gap distance is monitored and adjusted continuously so there’s no mechanical wear and no shear forces on the blood. The double-sided impeller is the single moving part in the pump, and is driven by what looks very much like the stator coils of a brushless DC motor.
One of the big advantages of the BiVACOR TAH is that it’s far smaller than the current crop of TAHs. That’s important because the space available in the thoracic cavity is quite limited, even in large male patients. Female patients and children, who tend to have smaller hearts, are often difficult to fit with a TAH. The BiVACOR heart also ticks the box of less intrusive external power; electrical cables are much easier to route into the chest cavity and less likely to act as a conduit for infection. It’s also quite possible that advances in battery technology will make implantable power sources possible in the near future; coupled with transdermal inductive charging, the BiVACOR heart and those like it could be the first practical permanent total artificial hearts.
There’s clearly more to do here; some basic questions, like does the human body have a physiological reason for a pulse, remain to be answered. Luckily, the BiVACOR heart can be programmed to provide pulsatile flow, so they’re well-positioned no matter what the answer is. But the fact that we don’t even know the answer yet shows how far we have to go. Luckily, the engineering appears to be catching up to where it needs to be for a permanent artificial heart to finally become a reality.
0 Commentaires