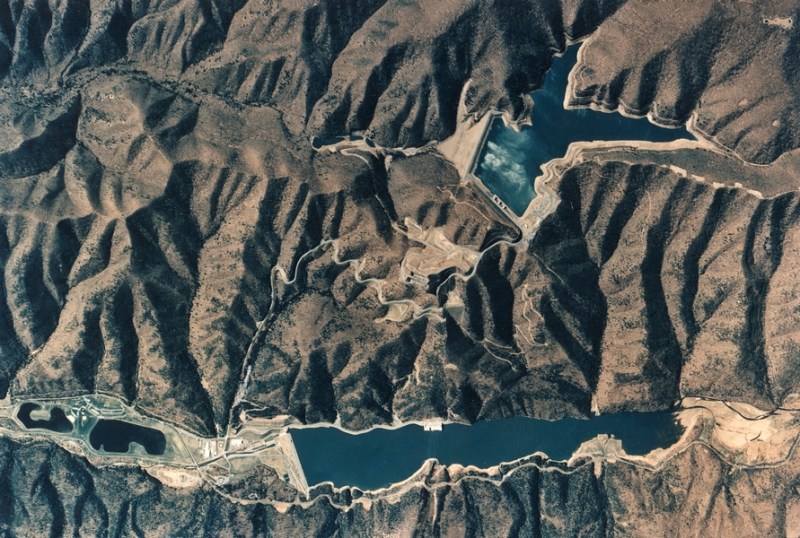
Although every electrical grid begins with the production of electricity, there are times when storing this power in some form instead of using it immediately is highly convenient. Today’s battery-powered gadgets are an obvious example of such time-shifting, but energy storage plays a major role on the grid itself, too, whether in electrochemical, mechanical or in some other form.
Utility-level energy storage is essential for not only stabilizing the grid, but also to time-shift excess energy and provide a way to deal with sudden spikes in demand (peak-shaving) plus demand drops by absorbing the excess energy. The health of the grid can essentially be regarded as a function of its alternating current (AC) frequency, with strong deviations potentially leading to a collapse of the grid.
Naturally, such energy storage is not free, and the benefits of adding it to the grid have to be considered against the expense, as well as potential alternatives. With the rapid increase of highly volatile electrical generators on the grid in the form of non-dispatchable variable renewable energy, e.g. wind turbines and PV solar, there has been a push to store more excess power rather than curtailing it, in addition to using energy storage for general grid health.
Types Of Storage
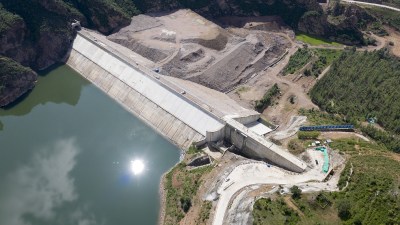
As mentioned, the biggest threat to grid stability comes in the form of loss of grid frequency, as this indicates a situation where connected generators and other inputs are no longer able to synchronize. The result of this is generally a cascading failure blackout which requires a slow and painful restart of the system to recover from. For this reason it is essential that on-grid storage is available that can respond to spikes and drops in demand so that supply and demand can be constantly adjusted.
These increases and drops in demand are highly dynamic, often requiring a response from an energy storage system within a matter of milliseconds, while other demand changes are more gradual and spaced out over the course of minutes to hours. For the fastest possible response, flywheel and battery storage are highly suitable, while pumped hydroelectricity storage (PHS) and compressed air energy storage (CAES) are suitable for more gradual ramp-up of energy absorption and release over longer periods of time.
When it comes to these grid stabilization systems, the actual cost of the energy is of secondary importance, as their primary function is to prevent the grid from collapsing. In that regard such storage systems are an integral part of the grid. This is different from the way that ‘grid storage’ has entered the public vernacular, in the sense of time-shifting large amounts of energy produced by renewable energy sources such as wind turbines and PV solar panels.
At its most extreme, a national grid is envisioned that uses nothing but variable renewable energy as well as hydroelectricity, while storing excess energy in grid storage to allow for time-shifting and release over the span of weeks to months as supply fluctuates. Naturally, in this scenario where dispatchable generators like thermal plants are replaced with mostly non-dispatchable sources and grid storage, a number of factors are essential. First is the system cost, followed by the operating cost, as these determine the price tag added to any energy released by these storage solutions.
To scale energy storage to the required levels for this scenario, technologies that can match PHS and CAES in terms of system and operating cost are required. For decades, reflow flow batteries have been developed as a possible solution.
Redox Flow Batteries
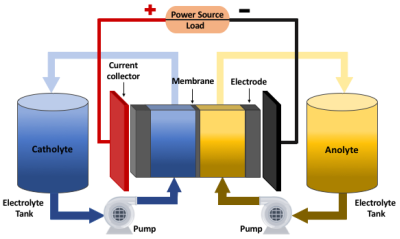
A redox (from reduction-oxidation) flow battery – or RFB – is a type of electrochemical battery that uses a liquid anode and/or liquid cathode electrolyte. In most implementations, the liquids for for the cathode and anode are separated by a membrane which allows for charges to be exchanged. Theoretically this kind of system would allow for a very large energy storage capacity along with most of the benefits of non-flow batteries such as fast charge and discharge response times.
Unfortunately, despite decades of research, even the most promising type of flow battery, vanadium-vanadium, has very low specific energy of ~20 Wh/kg of electrolyte where old-school lead-acid cells manage 25-50 Wh/kg and lithium-ion batteries over 200 Wh/kg. This means that the electrolyte tanks have to be quite large to match the energy capacity of e.g. a Li-ion or lead-acid based battery storage system. The density limit here is set by the ability to dissolve the redox compounds into the solvent, which for all-vanadium flow batteries is generally sulfuric acid (H2SO4).
These and other issues with flow batteries in general were covered by Clemente et al. (2020). Among the issues covered are also longevity, particularly with the membrane material, but also with the electrolyte itself due to unwanted reactions with hydrogen and oxygen. As flow batteries rely on electrolyte flow, the flow characteristics of the electrolyte, as well as the energy spent on pumping this electrolyte around and getting the optimal flow rate add engineering complications to what at first glance would seem to be a straightforward system.
Another such complication concerns the difficulty with determining state of charge. Since the voltage on the cell’s electrodes does not correspond to how much of the redox couple species has been converted to the charge/discharge state. With vanadium flow batteries, one option here is to estimate using the color of the electrolyte, as the redox process changes the physical properties of the vanadium species. For full automatic charge control, these are but many of the engineering questions.
As noted by Xu et al. (2018), the system efficiency of an flow battery is >60%, with ~82% possible with improvements in an all-vanadium batteries. An unfortunate aspect of an flow batteries are the parasitic losses due to the electric pumps, in addition to ohmic losses and flow resistance losses. As the redox reaction is exothermic, some energy will inevitably be lost during operation.
Fully charging a flow battery implies having all of the redox couple species undergo the desired redox operation. Because this involves interactions at the membrane surface separating the two electrolyte flows, the likelihood of this decreases with the charge state as fewer redox couples will interact. Increased electrolyte flow compensates for this to some extent, but this likely increase the parasitic losses, and makes simply scaling up the system by increasing the tank size unattractive.
When compared to the much higher round-trip efficiency and lower complexity of e.g. Li-ion batteries (>90%), it should come as little surprise that most large-scale grid-level battery solutions in commercial operation today employ such Li-ion cells.
The Cost Picture
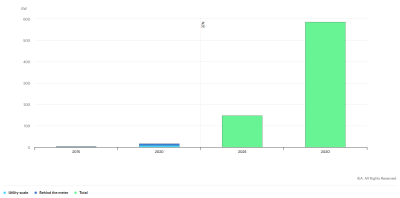
In the current applications of grid-storage, not an enormous amount of capacity is needed. With a few megawatts of strategically placed flywheel- and battery-based systems enough capacity should be available to respond to shifts in demand with some limited time-shifting.
For long-duration (~4 hours) storage, CAES and PHS are highly preferred where the environment allows for them to be used. A common use of such long-duration storage systems involves storing the energy produced by thermal power plants in times of low demand. Thermal plants often have optimal operating levels which makes it cost-effective to time-shift excess power for later discharge.
With more non-dispatchable sources being added to the grid storage capacity has to be increased for time-shifting like never seen before, along with the entire electricity market business model. Whereas thermal plants and hydroelectrical plants sell the electricity they produce on the capacity market using multi-year contracts, variable renewable energy installations sell the produced electricity on the energy market, which means the power they produce is opportunistic and unpredictable. Essentially electricity is sold to the grid when it’s available, regardless of whether it is needed at that point in time or not.
What this means is that for successful grid integration, these systems require significant amounts of spinning reserve to compensate for drops in supply, and storage of excess power as detailed in e.g. the Net Zero scenario projection by the IEA, which sees the current 10 GW of utility-level storage (in addition to 7 GW behind the meter) increase to 600 GW by 2030.
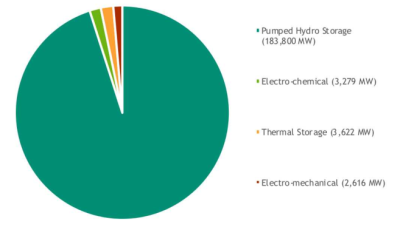
In a 2013 analysis by Purdue University’s State Utility Forecasting Group, the projected cost for a number of storage solutions were compared. Noted as an essential point are the system costs over time, which in the case of both PHS and CAES are quite minimal, since these systems tend to require relatively little maintenance while lasting many charge/discharge cycles. At the time Li-ion batteries were not a big player in the energy storage market.
In a more recent analysis by Sandia National Laboratories from 2018, we can see that the overwhelming majority of grid-storage is PHS (>183 GW). Of battery storage in the US in that year, Li-ion already made up 78% of installed battery storage, a number which has grown by a number of GW since, for example with Vistra’s 1.2 GWh (300 MW) Li-ion facility at Moss Landing, Monterey County, California, and its subsequent Phase II extension. Although both installations are currently offline, having caught fire, showing an inherent risk with battery storage.
According to the US Energy Information Agency (EIA), US power usage will reach about 4,000 TWh in 2023, which gives some indication of the challenge if a significant part of the world’s electricity needs are to be met from utility-level grid storage. Taking the Vistra Moss Landing storage as example, to cover 1 TWh (0.025% of the US demand), 625 of these 1.6 GWh facilities would be required, sufficient to cover the nation’s demands for a brief while. This lack of large-scale, long-duration storage is also noted in the Sandia National Laboratories report.
The Environmental Impact
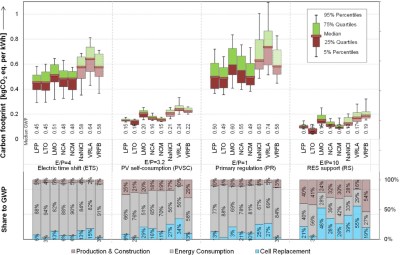
It seems clear that at least for now Li-ion battery systems are taking the spoils with grid storage since both PHS and CAES cannot be reasonably expanded on account of their limitations with regard to geology and similar. In addition to the higher costs of Li-ion, an important consideration becomes the environmental cost of these batteries, as the manufacturing of batteries is energy intensive and produces significant amounts of greenhouse gases (Baumann et al., 2016).
With the addition of such battery systems, the effective carbon emissions of variable renewable electricity can reach levels of around 100 gCO2e/kWh when lifetime emissions are taken into account. When considering the economical cost and environmental impact of carbon emissions, this would thus seem to be rather self-defeating from an environmental point-of-view, not to mention costly.
Using dispatchable hydroelectricity, coal, gas and uranium the conversion from gravity potential, carbon or fissile matter into electricity is performed on-demand. In comparison using mostly intermittent sources for powering a nation’s grid would seem to be an unsolved problem as this requires storing electricity in enormous amounts. Although a reasonable solution may be found in the future, at least at this point in time there is no mature, scalable technology that can perform this function in a way that could be considered economical.
[Heading image: Bath County Pumped Storage Station (Credit: CHA)]
0 Commentaires