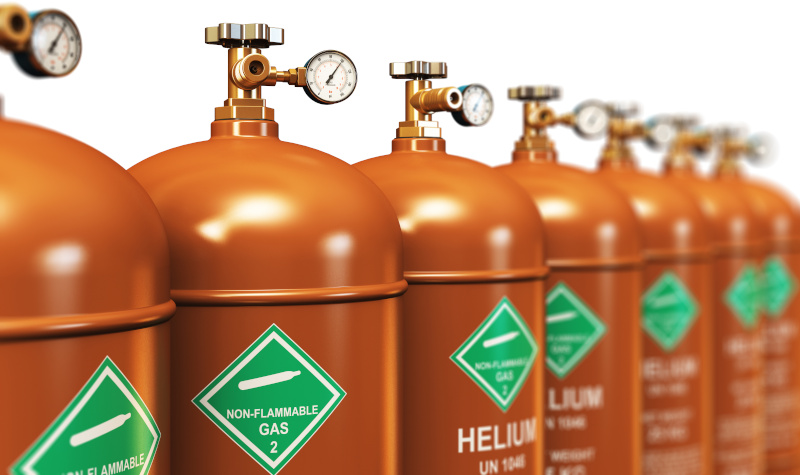
With a seemingly endless list of shortages of basic items trotted across newsfeeds on a daily basis, you’d be pardoned for not noticing any one shortage in particular. But in among the shortages of everything from eggs to fertilizers to sriracha sauce has been a growing realization that we may actually be running out of something so fundamental that it could have repercussions that will be felt across all aspects of our technological society: helium.
The degree to which helium is central to almost every aspect of daily life is hard to overstate. Helium’s unique properties, like the fact that it remains liquid at just a few degrees above absolute zero, contribute to its use in countless industrial processes. From leak detection and welding to silicon wafer production and cooling the superconducting magnets that make magnetic resonance imaging possible, helium has become entrenched in technology in a way that belies its relative scarcity.
But where does helium come from? As we’ll see, the second lightest element on the periodic table is not easy to come by, and considerable effort goes into extracting and purifying it enough for industrial use. While great strides are being made toward improved methods of extraction and the discovery of new deposits, for all practical purposes helium is a non-renewable resource for which there are no substitutes. So it pays to know a thing or two about how we get our hands on it.
A Product of Decay
Despite the fact that it’s the second most abundant element in the visible universe, helium is surprisingly rare on Earth. While it was first discovered in spectrographs from the sun and other stars in the 1860s, getting enough helium to study and make the determination that it’s an element would wait another 30 years, when a gas with the same spectral signature was released by dissolving a sample of uranium ore in acid.
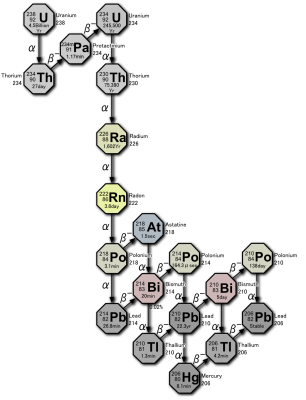
The discovery of helium on Earth came at an opportune time in the history of chemistry. The late 1800s and early 1900s saw the focus of chemistry expand from reactions involving atoms as a whole to the subatomic realm, on the level of the electrons, protons, and neutrons that make up atoms. Radioactivity had just begun to be explored, and the existence of alpha, beta, and gamma rays was already known at the time helium was first isolated. And so when Rutherford and Boyd discovered that alpha rays are actually particles consisting of two protons and two neutrons, which is identical to the nucleus of a helium atom, it immediately suggested a mechanism for how helium managed to become trapped within uranium ore.
Like all heavy radioactive elements, uranium decays along a specific series of elements. The Uranium Series starts with the isotope 238U, the naturally occurring and relatively abundant isotope of uranium. 238U has a half-life of about 4 billion years, and when it decays, it does so by releasing an alpha particle. The loss of a pair of protons and a pair of neutrons turns the 238U into 234Th, or thorium-234. The liberated alpha particle, which is really a helium nucleus, easily soaks up two electrons when it is absorbed by pretty much any matter it runs into, creating an atom of helium.
This neatly explains why helium was inside that sample of uranium ore — over time, uranium decay had released alpha particles that were absorbed by the rock, gaining the electrons needed to become helium atoms. Helium accumulated over time, collecting in the rock’s pores, only to be liberated when the minerals in the rock were finally dissolved. And this same process, albeit on a geological scale, is the key to industrial helium production.
A Gas in a Gas
Unlike most industrial gasses, helium is not present in the atmosphere in any significant concentration. Any helium that isn’t somehow sequestered after it is produced will find its way into the atmosphere and quickly be lost, rapidly ascending to the upper atmosphere and eventually out into space. So isolating helium from air as we do for oxygen, nitrogen, argon, and other gasses is not practical. Rather, we need to look under our feet for significant reservoirs of helium.
Luckily, the same geological conditions that tend to trap natural gas in underground reservoirs also tend to trap helium, and so natural gas wells are the biggest source of helium. Historically, the United States has been the main supplier of helium to the world’s markets, with most coming from natural gas wells in Oklahoma, Kansas, and Texas. Here the gas coming out of the ground is up to 7% helium, which is more than enough for profitable extraction.
Natural gas is a mixture of methane, nitrogen, carbon dioxide, and higher gaseous alkanes like ethane and propane. Where sufficient helium is mixed in — anything above 0.4% is considered profitable — extraction and purification of the helium are performed by fractional distillation. Helium has the lowest boiling point of any element, meaning that every other gas can be isolated by dropping the temperature and controlling the pressure.
The first step in helium production is to scrub any CO2 and hydrogen sulfide (H2S) from the natural gas. This is done in an amine treater, where the chemical monoethanolamine (MEA) is sprayed into the gas stream inside a reaction vessel. The MEA ionizes the acidic compounds and makes them soluble in water, allowing them to be scrubbed from the natural gas. The scrubbed gas is pretreated further by passing it over a molecular sieve, such as zeolite, and a bed of activated carbon, to remove water vapor and any of the heavier hydrocarbons.
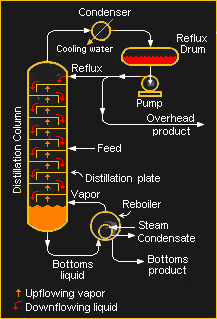
What’s left after these pretreatment steps is mainly methane and nitrogen, but also some neon and helium. The gas is cooled by passing it through a heat exchanger, then through an expansion valve into a baffled fractionation column. The sudden drop in pressure lowers the temperature of the gas enough that the methane, which boils at -161.5°C, condenses into a liquid and drains to the bottom of the column.
The remaining gas, now mostly nitrogen and helium, is passed through a condenser that cools the stream even further. When the temperature of the mixture falls below -195.8°C, the nitrogen condenses out as a liquid. Along with the liquid methane, the liquid nitrogen is piped to the heat exchangers that were initially used to cool the incoming pretreated process gas. The now-gaseous nitrogen and methane, valuable products both, are piped to storage tanks.
About half of the remaining process gas is helium, with the rest being a mixture of contaminating methane and nitrogen, along with a little bit of hydrogen and neon. This mix is called cold crude helium, and must now undergo further purification to get to the purity level required for industrial use. Purification begins with another heat exchanger that drops the crude helium mix below the boiling point of nitrogen, to condense out the remaining nitrogen and methane contaminants. This step takes the crude helium to about 90% purity.
Final Purification
To get rid of the hydrogen, oxygen is introduced and the mix is heated in the presence of a catalyst. The hydrogen and oxygen form water, which can be separated out of the process gas stream before it heads to final purification by pressure swing adsorption, or PSA. Pressure-swing adsorption is the same process used in oxygen concentrators, including many of the DIY versions we’ve seen as a response to COVID-19. PSA uses the ability of materials known as molecular sieves to selectively adsorb a gas. In helium purification, the 90% pure gas is pumped into a pressure vessel containing a molecular sieve, usually Zeolite. The contaminating gasses are preferentially adsorbed into the Zeolite, leaving the output stream nearly pure helium. When the first column is saturated with contaminants, flow is switched to a second column that had been previously regenerated by backflushing it with pure helium. The gas flow switches back and forth between the two columns, one purifying the helium while the other is regenerated. The result is gaseous Grade-A helium at 99.995% purity.
The process described here is by no means the only way to extract helium from natural gas, but it does represent the most common way of producing the gas, mainly because most of the pretreatment and initial purification steps are already used to process natural gas for fuel and as a feedstock for the chemical industry. Other methods include a completely PSA process, which can use natural gas with a mere 0.06% helium concentration, and membrane separation, which relies on the fact that helium can penetrate a semipermeable membrane much easier than the much larger methane and nitrogen molecules. Membrane separation technology can be much more energy-efficient than traditional fractional distillation, since it doesn’t require phase changes and the energy they require.
But Are We Running Out?
Knowing the abundance of uranium-238 in the Earth’s lithosphere along with its half-life, it’s possible to estimate the amount of helium produced by the radiogenic process. It turns out to be not a lot — only about 3,000 metric tons a year. And almost all of that escapes into the atmosphere and out into space. So in much the same way as the natural gas in which it is usually found, helium is effectively a non-renewable resource.
But does that mean we’re running out? Yes, like any other limited resource, eventually we’ll extract all there is to extract. But that doesn’t necessarily mean we’ve found all the helium there is to find. Exploration has led to new deposits in the United States, and massive helium finds in places like Algeria, which became the second-largest helium producer in the world in the early 2000s. Qatar also had a huge helium find in 2013, moving it up to second place worldwide. These finds, along with the recent discovery of natural gas wells in South Africa with up to 12% helium, promise to address some of the concerns about losing access to this irreplaceable gas.
But at the end of the day, these new finds only push back the clock and forestall the inevitable day when the helium finally runs out. We may catch a break if commercial-scale fusion ever becomes a thing, but that breakthrough has been “only twenty years away” for the last 80 years.
0 Commentaires