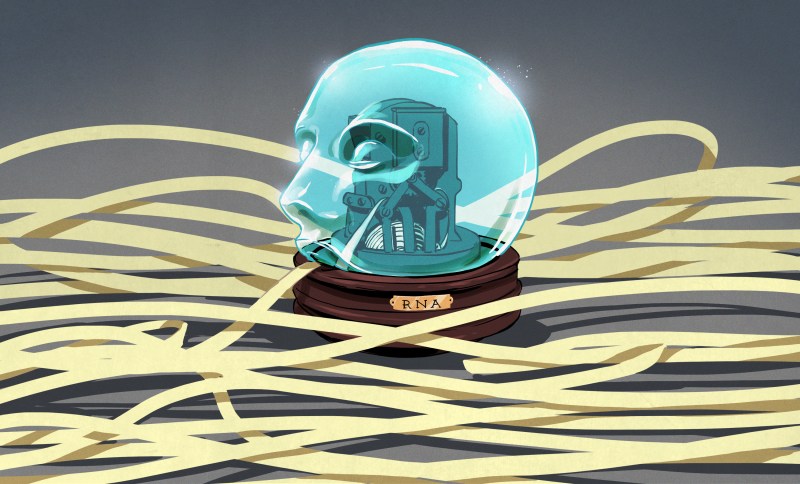
Before the SARS-CoV-2 pandemic took hold, few people were aware of the existence of mRNA vaccines. Yet after months of vaccinations from Moderna and BioNTech and clear indications of robust protection to millions of people, it now seems hard to imagine a world without mRNA vaccine technology, especially as more traditional vaccines seem to falter against the new COVID-19 variants and the ravages of so-called ‘Long COVID’ become more apparent.
Yet, it wasn’t that long ago that Moderna and BioNTech were merely a bunch of start-ups, trying to develop profitable therapies for a variety of diseases, using the brand-new and largely unproven field of RNA therapeutics. Although the use of mRNA in particular for treatments has been investigated since 1989, even as recently as 2017 there were still many questions about safe and effective ways to deliver mRNA into cells, as per Khalid A. Hajj et al.
Clearly those issues have been resolved now in 2021, which makes one wonder about the other exciting possibilities that mRNA delivery offers, from vaccines for malaria, cancer, HIV, as well as curing autoimmune diseases. How did the field of mRNA vaccines develop so quickly, and what can we expect to see the coming years?
Vaccines in a Nutshell
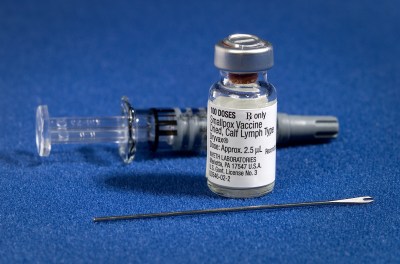
Before there were vaccines, the practice of inoculating was quite common, especially in China, where references dating back to at least the 15th century have been found. Focusing mostly on smallpox, this so-called variolation (smallpox inoculation, derived from the name of smallpox, Variola) involved taking live pathogen from a smallpox patient and introducing it into someone without pre-existing immunity to the disease.
When Edward Jenner popularized the practice of using cowpox instead of smallpox in the 18th century to induce immunity against the latter, this was the beginning of vaccination (Latin vaccinus, ‘of or from the cow’). Unlike inoculation, vaccination seeks to use a heterotypical pathogen (like cowpox), inactivated or weakened pathogens, or specific elements (units) of a pathogen which can induce immunity against that pathogens.
At the core of the human immune system lies the concept of antigens, which are specific proteins or other elements of a pathogen or toxin which the immune system can recognize using antibodies. These antibodies are Y-shaped proteins that can bind to specific antigens. They can be either attached to B cells (B cell receptors, BCRs), or free-floating in extracellular fluids (e.g. blood plasma).
With natural immunity from infection by a pathogen, the body’s adaptive immune system usually learns to recognize the foreign antigens and deal with the threat. The use of a vaccine is to present the immune system with these antigens (e.g. the SARS-CoV-2‘s distinctive spike protein) so that the immune system (specifically B cells) gets a chance to memorize it so that it can respond much sooner to an infection.
Vaccines Without the Complexity
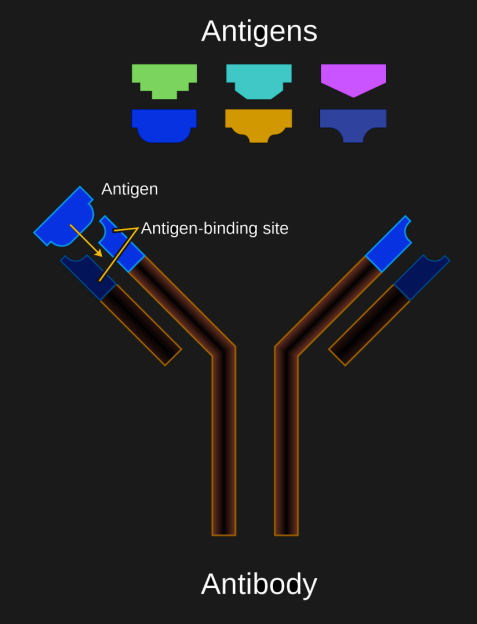
As the main point of a vaccine is to present the immune system with the most effective antigens, this has led to a wide range of vaccine types over the years that seek to optimize the immune response. Here the rapid development of SARS-CoV-2 vaccines has been highly instrumental in illustrating the state of the art. Essentially, just two types of vaccines were able to be developed in the minimal amount of time available: viral vector and mRNA vaccines.
Both of these are new types of vaccines and neither have been deployed on this scale. Where they differ from other types of vaccines is that they do not require that the pathogen is grown outside of the body, but instead they focus on delivering genetic material (mRNA) into cells where these can be translated by the cell’s ribosomes into the proteins that form the target antigen.
In theory, this would skip a number of complexities, as it only requires that enough of the RNA strings are produced and inserted into the body’s cells, rather than needing to synthesize the target antigens (proteins) and inject those. In the case of traditional vaccines which use whole pathogens, the use of RNA skips the risk of accidental outbreaks caused by these live pathogens, including attenuated strains as seen commonly with polio vaccines (vaccine-induced polio).
For all their similarities, however, mRNA vaccines have a major advantage over viral vectors: the use of a lipid nanoparticle-based (LNP) delivery system which stabilizes the fragile mRNA string. This was first used with Patisiran, an RNA therapeutics medication that uses siRNA (gene-silencing RNA) to treat polyneuropathy. Through careful design of these LNPs, they are effectively as unremarkable to the immune system as the (lipid-based) membranes of the body’s own cells.
Since viral vectors by definition use viruses for breaking-and-entering into cells, these viruses are highly likely to be detected by the immune system, and some may have antigens which are already known to the body. An mRNA vaccine’s LNP coating makes it comparatively stealthy. Another major issue with viral vectors is that they require modification of the viral RNA, inserting the RNA string that needs to be carried as payload, while ensuring that these genetic modifications were done correctly.
Spiking Those Cells
Once an mRNA vaccine is injected into the body, the vast majority of the LNP-coated particles are absorbed by dendritic cells, which roam the extracellular space and can be regarded as the sentinels of the immune system. Within the dendritic cell the mRNA string is released, where it is ultimately processed by the ribosomes as any other mRNA string that originates from the cell’s nucleus. This results in the production of e.g. the SARS-CoV-2 spike protein, which the dendritic cell will then expose on its membrane to B and T cells.
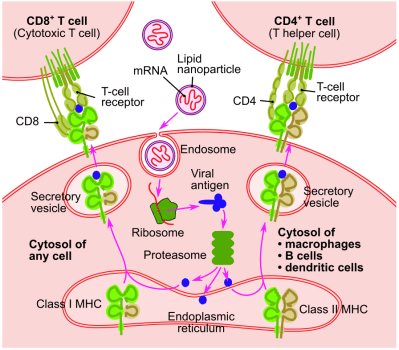
Important to note here is that these are not the exact same mRNA strings that would originate from the nucleus, as the cell’s defensive systems monitor the intrusion of foreign RNA. This is circumvented through nucleoside-modified mRNA (modRNA), in which nucleosides are swapped for synthetic analogs. This does not affect the handling by the ribosomes. In the case of CureVac‘s SARS-CoV-2 mRNA vaccine, the use of unmodified mRNA strings is likely the reason why in trials it showed very low efficacy that currently prevent it from being a viable vaccine.
Based on the data, especially from the Moderna and BioNTech-Pfizer SARS-CoV-2 mRNA vaccines, we can deduce that the combination of the LNP coating and modRNA is what makes it as effective as it is. Another interesting recent finding by Jackson S. Turner et al. was that these mRNA vaccines also trigger B cells into forming germinal centers, which are effectively training grounds for B cells that promote a long-term memorization and thus immunity to a specific antigen.
Addressing Autoimmune Conditions
When it comes to disadvantages of an adaptive immune system, one can definitely point to autoimmune conditions. These involve T and B cells produced by the body which react to self-proteins, meaning the proteins that normally make up one’s own body. Autoimmune conditions range from type 1 diabetes to lupus, each involving a specific type or types of self-proteins that are attacked by our own immune systems.
Normally, after T and B cells are formed in the bone marrow, their cell receptors are randomly arranged through V(D)J recombination to give them the widest possible range. Central tolerance — negative selection of T and B cells — exists to tests each of these new lymphocytes to see whether they self-react and thus are likely to be involved in autoimmunity. Any T or B lymphocyte which responds to the presented self-proteins are terminated via apoptosis (programmed cell death) or are reprogrammed to not self-react any longer.
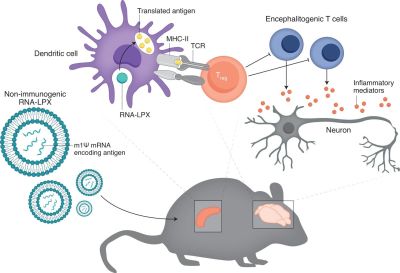
Although central tolerance, along with peripheral tolerance exist to prevent the possibility of autoimmune disorders while giving the best possible protection against new pathogens, there are many factors which can derail this, including a mutation in the autoimmune regulator gene (AIRE) and in the case of type 1 diabetes a mutation in the INS (insulin) gene.
Christine M. Wardell et al. describe a study by Christina Krienke et al. of a treatment for multiple sclerosis using a tolerizing vaccination approach. In mice, this treatment essentially cured an MS-like condition in the mice that were injected with this vaccine. Through a process of what could be called exposure therapy, the autoimmune response is downregulated and might conceivably be reset.
Related to this research is that of reducing or eliminating the immune response to harmless allergens such as pollen. As detailed in e.g. a literature review by Sandra Scheiblhofer et al. from 2018, which references both human and animal studies. These studies show that mRNA vaccines could hold the promise of a life-long reprieve from allergies as well.
Vaccine-To-Go
The good news around mRNA-based vaccines and RNA therapeutics is very hard to ignore at this point. The effectiveness of being able to essentially print strings of modRNA for a desired protein is hard to underestimate, along with the implications this has for e.g. cancer therapy. Both BioNTech and Moderna are involved in clinical trials that seek to assess whether customized mRNA vaccines that target the specific neoepitope antigens of a patient’s cancer can provide an effective therapy if not a cure for various types of cancers.
A recent paper by Katherine L. Mallory et al. covers a potential mRNA-based malaria vaccine. Encoding the PfCSP coat protein of the malaria parasite, initial findings show a sterilizing (i.e. no potential for transmission to other hosts) protection against the disease. If an effective vaccine could be developed based on this, it could save over 405,000 deaths yearly and countless millions of malaria cases.
In another paper that was published this year by Zekun Mu et al., the progress and future paths of an HIV mRNA vaccine are explored. Such vaccines would provide a layered response, initially eliminating as many of the HIV pathogens as possible, followed by a secondary, cytotoxic response that seeks to eliminate any cells which have become reservoirs for HIV. While still early days, there is a lot of promise here too.
The Holy Grail of Immunotherapy
Probably the most attractive aspect of RNA therapeutics is that it forms essentially the best form of immunotherapy, by working directly with the immune system. By reinforcing or silencing gene transcription, certain deficiencies and excesses can be directly addressed, while mRNA vaccines seem to hold the promise of both up- and downregulating the immune response.
In this way it works in harmony with the body’s natural systems, while having the power to provide corrections and information as necessary. Simultaneously, considering the ease and relatively low cost of customized mRNA vaccines, this might mean that even orphan diseases might finally get some much-needed attention. This might make RNA therapeutics and mRNA vaccines probably one of the biggest medical revolutions since the discovery of antibiotics.
Although there were concerns last year about the ultra-cold storage requirements for mRNA vaccines, as we learn more about mRNA vaccines and their stability over time, we now know that we can forget about them in the back of a regular refrigerator for a month without any worries. This should help with making mRNA vaccines and RNA therapeutics the norm over the coming years.
(Heading image: Schematic working of mRNA translation by a ribosome with help from tRNA)
0 Commentaires