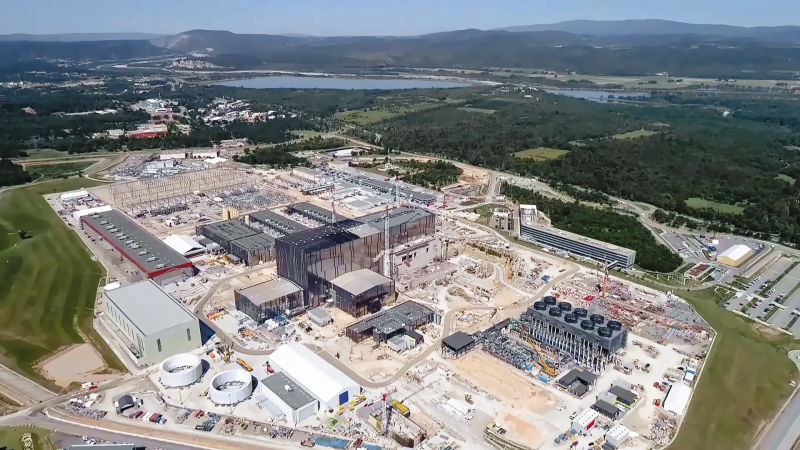
Doing something for the first time is tough. Yet to replicate the nuclear fusion process that powers the very stars, and do it right here on Earth in a controlled and sustained fashion is decidedly at the top of the list of ‘tough’ first times. What further complicates matters is when in order to even get to this ‘first’ you also add in a massive, international construction project and a heaping of geopolitics, all of which is a far cry from past nuclear fusion experiments.
With the International Thermonuclear Experimental Reactor (ITER) as the most visible part of nuclear fusion research, it is perhaps little wonder that the recent string of delays and budget increases is leading some to proclaim doom and gloom over the entire sector. This ironically in contrast with the recent news from the US’s NIF and its laser-based inertial confinement fusion, which is both state-funded and will never produce commercial power.
In light of this, it feels pertinent to ask the question of whether ITER is the proverbial white elephant, or even the mausoleum of international science that a recent article in Scientific American makes it out to be. Is fusion research truly doomed to peter out amidst the seemingly never-ending work on ITER?
History Sometimes Rhymes
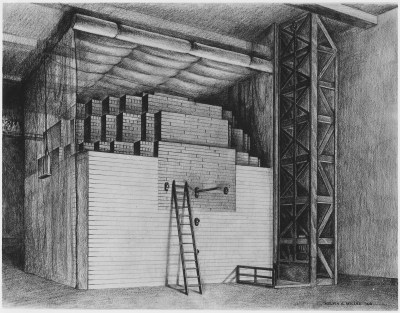
What sometimes trips people up is that although both nuclear fission and nuclear fusion pertain to energy obtained from interactions between atoms, only one of these is exceedingly easy to make happen. All that it takes for nuclear fission to occur is for enough fissile material (such as uranium-235) to exist in the same general location, and enough neutrons to zip about that allow for a nuclear chain reaction to begin and sustain itself. This is why natural nuclear fission reactors existed during the formative years of the Earth, when amounts of fissile material in the Earth’s crust was still relatively high.
It is little wonder then that replicating this process during the 1940s in graphite pile reactors was a relatively straightforward process: the graphite moderates the emitted neutrons, which are thus slowed down into thermal neutrons which cause more fission events, which produce more neutrons, which are moderated, etc. Although this obviously took some engineering to get all the details right, the first artificial nuclear fission reactor became a fact on December 2nd, 1942 when Chicago Pile-1 (CP1) sustained a nuclear chain reaction.
Although CP1 had no cooling, shielding or other amenities, CP2 and derived reactors were already usable as crude predecessors to the commercial power reactors that would form the backbone of the nuclear fleet build-outs in countries like Canada, the US, France and Japan during the 1970s, most of which reactors are still operating and producing power today. With this success in mind, one might be forgiven for being optimistic about the chances of also tackling nuclear fusion.
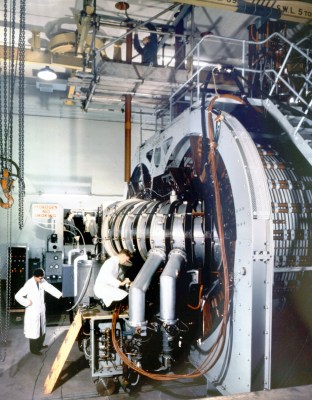
During the 1950s, the UK had become one of the leading forces behind nuclear fusion plants, with the research up till that point indicating that the Z-pinch (zeta pinch) method of containing deuterium-tritium plasma within a magnetic field was all that would be needed to create a commercial fusion power plant. Unfortunately, it was discovered that in the larger prototype Z-pinch reactors the plasma was not as well-behaved as originally assumed. Instead plasma instabilities would occur seemingly randomly within the reactor, which led to the near-demise of fusion research, until the invention of the tokamak breathed new life into the field.
These first tokamak designs with their donut-shaped field proved to be significantly more capable of dealing with the whims of the highly dynamic and very hot plasma as it churned within the confinement of the field lines. Ultimately, the demise of commercial Z-pinch-based fusion reactors proved to be not the end of the dream of commercial fusion, but rather the beginning of a much better understanding of plasma physics.
Many Eggs, Many Baskets
Of the things which have changed significantly over the past seventy-odd years, advances in computer technologies and materials science have allowed us to create reactor vessels and magnets that would have been deemed near-magical in those early days. In addition, using supercomputers we can simulate plasma fields in great detail, simulations which are continuously improved by experimental data obtained from the many active fusion research reactors around the world.
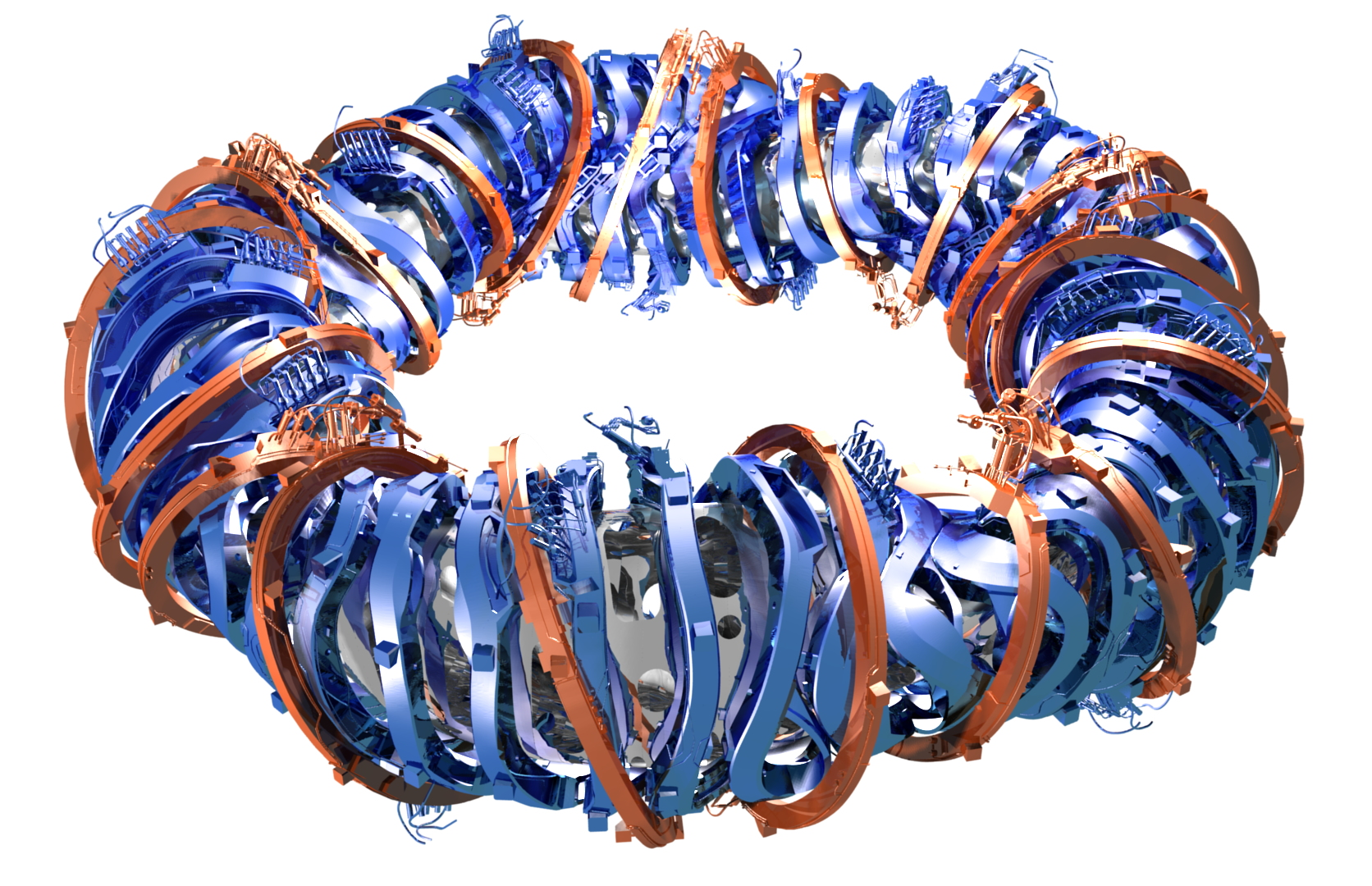
Perhaps most fascinating of these reactors are the stellerators, which are not a new concept, but which require a level of understanding of plasma field dynamics that was beyond the grasp of our understanding and simulation tools until a few decades ago. Since then, this type of fusion reactor has rapidly caught up on tokamak designs, with the Wendelstein 7-X being the most well-known. Using its oddly shaped electromagnets, it creates a magnetic field that doesn’t just trap and constrain the plasma as in a tokamak, but instead seeks to ‘flow’ with the plasma. Theoretically this will increase efficiency, and allow for continuous operation to demonstrate the feasibility of stellerators for use in commercial power plants. This particular stellerator has most recently been updated to its final configuration with cooled diverters, which should allow it to demonstrate a continuous run with hot plasma for up to half an hour.
Meanwhile, a mixture of both state-financed and private fusion reactors are vying for the spotlight, with the UK’s JET tokamak and ST40 spherical tokamak getting headlines, and the private Commonwealth company putting decades of US national laboratory fusion research to good use in its innovative ARC and SPARC reactor designs that employ high-temperature, superconducting electromagnets. Of the remaining research reactors, the Chinese fusion program is probably the most ambitious, using their medium-size HL-2M tokamak in particular to not only provide experimental validation for ITER’s design, but also for the China Fusion Engineering Test Reactor (CFETR).
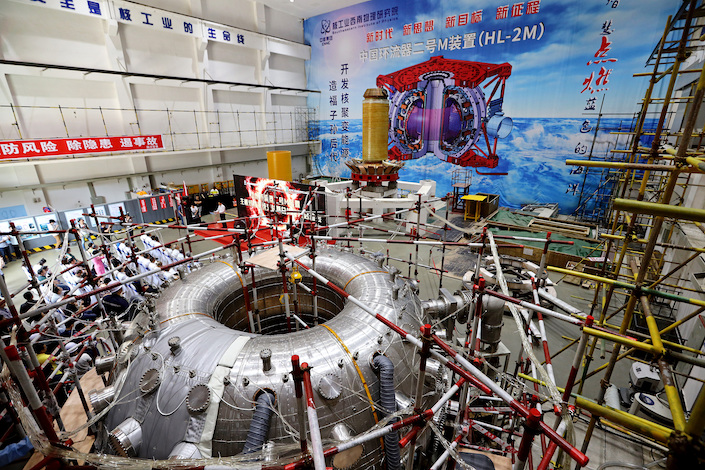
Whereas the HL-2M tokamak has a major radius of a mere 1.78 m, the CFETR as it is currently scheduled to be constructed this decade will feature a major radius of 7.2 m, which would make it larger than ITER at 6.2 m. Essentially, CFETR will fall between ITER and its intended DEMO successor, allowing it to validate DEMO principles, such as breeding tritium fuel from a lithium blanket inside the reactor vessel to sustain continuous operation.
Interestingly enough, according to the current schedule, the CFETR should begin operation around the same time that ITER is projected to begin fusion with deuterium-tritium fuel, around 2035. Despite the experimental nature of CFETR, China is looking at using the approximately 2 GW of thermal energy for electricity production, with molten salt as buffer. As these early fusion reactors are likely to need frequent breaks to cool down and for maintenance, having an intermediate buffer might already make fusion reactors viable power plants by next century.
Engineering Chops
As an international undertaking, ITER relies on the nations involved in its construction to provide the components for the tokamak, which appears to cause issues that mirror the troubles that plagued Boeing’s 787 airliner, with many subcontractors around the world being provided with incomplete specifications, along with quality assurance troubles. Whether it concerns an airplane or fusion reactor, smaller issues can quickly snowball into worse issues, especially when they’re not tackled early on. Add to this the massively disrupted supply chains in 2020 and beyond due to the pandemic, and it would have been more amazing if things had continued without delay.
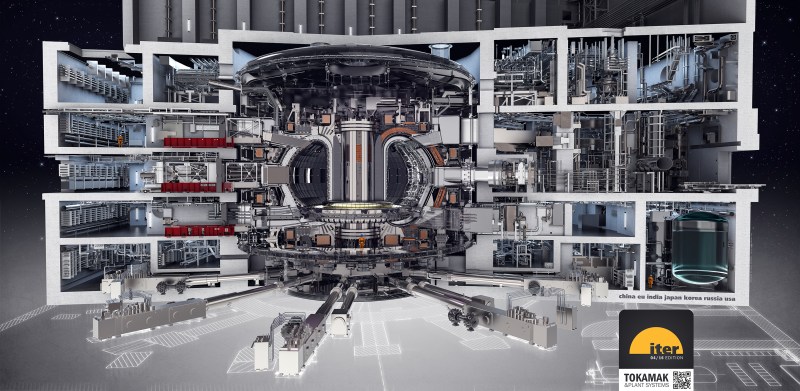
Although the experimental validation for aspects of ITER’s design continues using existing tokamaks, the actual assembly of the physical reactor has to be performed by skilled workers, who are provided with quality components, materials, and tooling. Reportedly, this is where ITER does have some major issues, with defective components arriving at the build site, and some workers claiming qualifications that they do not have. These are unfortunate project management aspects that are not exclusive to the construction of fusion plants.
In essence, then, it could be said that the ITER project has major issues that need to be addressed. Yet to mark ITER as somehow a monument to the folly of pursuing nuclear fusion power, or the final resting place of international scientific cooperation would be an offensively poor hot take on the state of the field. After all, the collective fields of plasma physics, fusion research and adjacent are cooperating every day for many decades now towards the singular goal of achieving sustainable nuclear fusion.
To then characterize ITER – with its roots in 1970s geopolitics – as somehow the end-all, be-all of fusion research is nothing if not dishonest. Whether or not ITER is ultimately finished next century and starts operating is more a question of sunk cost, but it is far from being humanity’s sole hope of unlocking fusion power, let alone the linchpin for international cooperation on the topic. Ideally the current issues with ITER will be resolved sooner rather than later, but it should not reflect on the field as a whole.
After all, ITER is just part of the puzzle, with private companies around the world investing in their own designs, multiple designs by nations like China with a strong nuclear industry courtesy of its ongoing fission plant build-out, and even Germany with its so far incredibly successful Wendelstein 7-X stellerator. All of whom are whittling down the last remaining issues towards making commercial fusion plants a reality.
(Heading image: Aerial view of the ITER site in 2020. (Credit: Macskelek) )
0 Commentaires